Computer simulations of copper indium gallium diselenide alloy (CuInxGa1-xSe2, or CIGS) suggest that indium segregation effects may play an important role in reducing the conversion efficiencies of photovoltaic/solar cell devices based on the material [Christian D. R. Ludwig et al, Physical Review Letters, vol105, p025702, 2010].
Despite these problems, the best CIGS solar cells have achieved efficiencies of 20.1%, although commercial devices fall somewhat short of this (up to ~14%). The theoretical maximum is around 30%. CIGS is one of the leading contenders for low-cost production of relatively high-efficiency cells.
The calculations were carried out by researchers at Johannes Gutenberg-Universität Mainz, IBM and Universität Duisburg-Essen. In fact, the lead author of the paper, Christian Ludwig, used a mainframe for his investigations that was recently donated to Mainz University by IBM as part of a Shared University Research (SUR) science award.
The starting point for the study was the observation that, despite an optimal bandgap for solar radiation absorption suggesting that a CIGS material with 70% Ga should yield the highest efficiency, in fact 30% Ga is experimentally found to be best. The estimate is based on the bandgaps of the non-alloyed materials CuInSe2 (1.0eV) and CuGaSe2 (1.7eV).
Indium segregation is a common problem for nitride semiconductors used to create green-blue-violet-ultraviolet light-emitting devices. Indium segregation refers to variations in the balance of indium and the other group III materials (such as gallium) in the alloy material.
The effect of indium segregation is not always negative – in some nitride light-emitting devices it is thought that the segregation effect creates localized states that can boost emission efficiencies in some cases. However, in the case of solar cells such effects are almost certainly detrimental, particularly in leading to fluctuations in the material's bandgap energy value.
The simulations used a hybrid of density functional theory (DFT), cluster expansion and Monte Carlo methods to simulate larger samples than usual with pure DFT. The cluster expansion is used to extract interaction energies from the DFT results that are used in the Monte Carlo of larger systems.
Dr Thomas Gruhn, head of the theory group involved in the work, explains the methods used: "Density functional calculations make it possible to assess the energies of local structures from the quantum mechanical point of view. The results can be used to determine temperature effects over wide length-scale ranges with the help of Monte Carlo simulations."
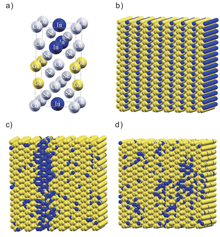
Figure 1. (a) CIGS unit cells showing positions of atoms in series of unit cells. Snapshots of: (b) a system of periodic unit cells; (c) the Ga-rich system at 348K; (d) the Ga-rich system at 406K (Ga atoms are yellow; In atoms are blue). Cu and Se are not displayed in the snapshots, and the size of the spheres is arbitrary.
The calculations ignore possible defects of the Cu and Se atom sites, concentrating on the effects of variations in the Ga/In site occupation in the crystal structure (Figure 1a). The formation energies of 32 CIGS structures were calculated using DFT methods. The Monte Carlo used supercells with up to 32 atoms with a simulation box measuring 16x16x8 (8192) active atoms. The temperature range of the simulations was between 290K (room temperature-ish) and typical CIGS process temperatures of 870K. The resulting inhomogeneity was assessed using cubic segments consisting of 16 atom sites. The standard deviation was used as a measure of inhomogeneity.
Two alloy systems were studied: 'Ga-rich' CuIn0.25Ga0.75Se2 and 'In-rich' CuIn0.75Ga0.25Se2. At low temperatures (290K) a histogram of the segment compositions show two peaks — one being around a few atoms of the non-rich atom, the other a small peak for the non-rich atom at around 15 (Figure 2). This is an indication of segregation with regions of unalloyed material — CuInSe2 (CIS) and CuGaSe2 (CGS). At higher temperatures, this behavior decreases and the alloy becomes more homogeneous.
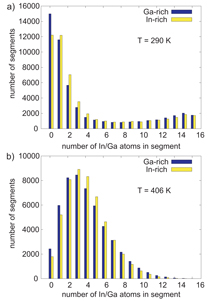
Figure 2. Histograms showing number of cubic segments in simulation box that contain 1–16 atoms of a certain type. Blue is the distribution of In atoms in Ga-rich CIGS, and yellow is the distribution of Ga atoms in In-rich CIGS at temperatures of (a) 290K and (b) 406K. A perfectly ordered In-rich or Ga-rich system would have 4 Ga/In atoms in every segment – the histogram would then have all entries in bin 4.
The segregation effect at low temperature is found to be worse for Ga-rich material, perhaps explaining the poorer performance of 70% Ga compared with 30% Ga CIGS solar cells. The mean value for both types is 4 non-rich atoms per box. The standard deviation is always higher for Ga-rich CIGS, again indicating higher inhomogeneity.
These results suggest that fast cooling of high-temperature processed material could minimize bandgap fluctuations, leading to better solar cell performance. The authors comment: "The lack of phase separation in actual solar cells shows that the In-Ga distribution is 'frozen' in a high-temperature state. Thus, higher production temperatures and a reasonably fast cooling will lead to better efficiencies, which has recently been shown experimentally."
In practice, such fast cooling has been limited by the heat resistance of the glass used as a substrate for CIGS solar cells.
This research forms part of the comCIGS project, which is looking to optimize CIGS solar cells with funding from the Federal German Ministry for the Environment, Nature Conservation, and Nuclear Safety (BMU). The project involves IBM Mainz and Schott AG, along with Johannes Gutenberg-Universität Mainz, the Helmholtz Center Berlin for Materials and Energy and Jena University.
To enable faster cooling of CIGS solar cells, Schott AG has developed a special glass with which the process temperature can be increased to well above 600°C. The cells that result are considerably more homogeneous, opening the way to cells with greater efficiency levels.
Gruhn reports: "We are currently working on large-format solar cells which should outperform conventional cells in terms of efficiency."
Mario Pedraza
Electrónica del Estado Sólido
Sección 2
Electrónica del Estado Sólido
Sección 2
No hay comentarios:
Publicar un comentario